#me article
Let’s look in detail at the process of boiling a pure substance.
We’ll start out with a compressed liquid. We’ll say it’s water at 25 deg C. Moreover, let’s say it’s contained in a cylinder with a piston so that the pressure inside the cylinder is always at atmospheric pressure.
If we start heating up the cylinder, the water inside will expand slightly. The pressure will remain constant as the piston moves with the water’s expansion. Once we get to 100 deg C, the water exists as a saturated liquid - any additional heat will cause it to vaporize.
If we keep adding heat to it, things get interesting. The water will start to boil. It’s volume will increase drastically, but its temperature will remain the same. All the energy you’re putting into it is going into the phase change. The amount of energy it takes to go from a liquid to a vapor is called the latent heat of vaporization. (Similarly, the amount of heat it takes for a solid to melt into a liquid is the latent heat of fusion.) So while the water in your cylinder is in the process of boiling, it exists as a mixture of saturated liquid and saturated vapor. Its temperature will remain at a constant 100 deg C throughout the process. Although its apparent volume will increase, its specific volume - the volume per unit mass - will also remain constant.
Once all the water has been vaporized, if you continue adding heat to the cylinder, the water will start to rise in temperature again and its specific volume will start to increase. In this state, it exists as a superheated vapor.
The entire process we just described looks like this.
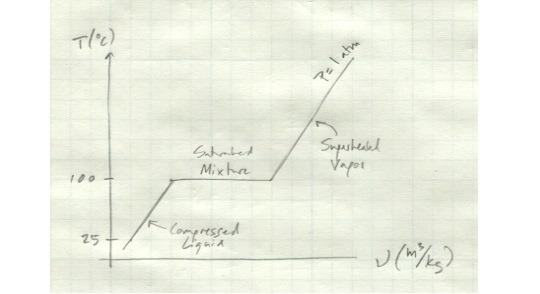
Note that this show temperature vs. specific volume for only one pressure - if we varied the pressure as well, things would look quite different. The interdependence of temperature, volume, and pressure will be important in our analysis of thermodynamic processes.
We’ve spent some time going over the basics of heat enginesandrefrigerators. These are machines which manipulate a working fluid through some sort of cycle in order to move heat from one location to another. We have some idea of the overall way they work, but to really understand the details of what’s going on, we’re going to have to know a little more about how this working fluid behaves.
For right now, we’ll restrict our discussion to materials which qualify as pure substances. A pure substance has the same chemical composition throughout - all its molecules are the same. We’re all familiar with phases of matter - solid, liquid, and gas are the ones we see on a daily basis. Matter in solid form has its molecules arranged in a regularly structured lattice. In a liquid, molecules have about the same distance from each other as they do in a solid, but they’re not held in a structure and can move around each other freely, although their close spacing means that intermolecular forces play a large part in their motion. In a gas, molecules are widely spaced and careen about as they please, interacting with each other only through collisions.
For thermodynamics-related purposes, we’re really interested most in liquids and gases. Controlling the transition between these two states is the key to moving a lot of energy around. So let’s define some terms.
Acompressed liquidorsubcooled liquid is one that is in no danger of vaporizing.
Asaturated liquid is one that is about to vaporize.
Asaturated vapor is one that is about to condense.
Asuperheated vapor is one that is not in any danger of condensing.
Distinguishing between these is important from a thermodynamics because a fluid will have different properties related to its ability to absorb and release energy in each state. In coming articles, we’ll look a little more closely at the exact process of transition between phases.
We’ve talked about the second law of thermodynamics as the idea that energy naturally flows from a point of high potential to low potential. There are a couple of other ways you can think about this.
One is called the Kelvin-Planck statement of the second law:
A device which operates on a cycle cannot exchange heat with a single heat reservoir and produce a net amount of work.
Compare this to the heat engine we looked at earlier. A heat engine schematically looks like this:
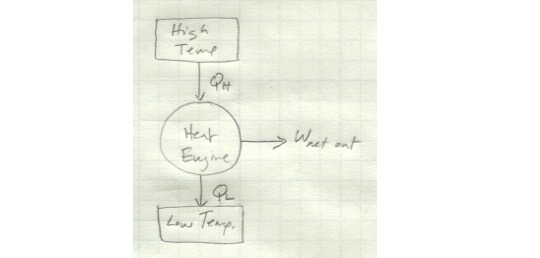
It exchanges heat with both a high- and low-temperature reservoir and produces a net amount of work. If instead it only exchanges heat with a single reservoir, it looks like this:
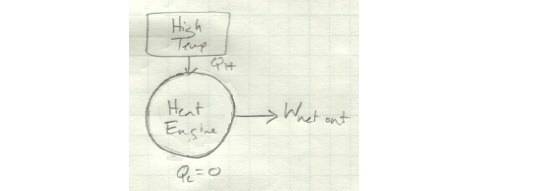
Looking at it like this, you see clearly why this is impossible: in this schematic, there is no Q_L - all of the heat coming in is converted to work. In other words, this device has 100 percent efficiency - something we know can’t happen.
Another formulation of the second law is the Clausius statement of the second law:
A device which operates on a cycle cannot move heat from a lower temperature reservoir to a higher temperature reservoir as its only effect.
This one also becomes clear if we look at it schematically. Here’s a regular refrigerator schematic:
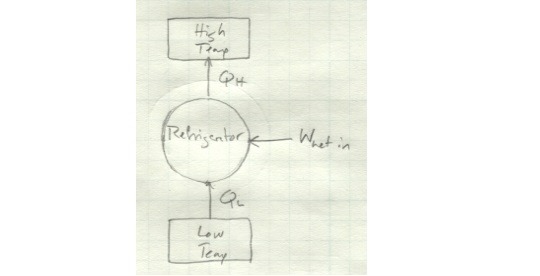
And here’s a refrigerator which violates the Clausius statement:
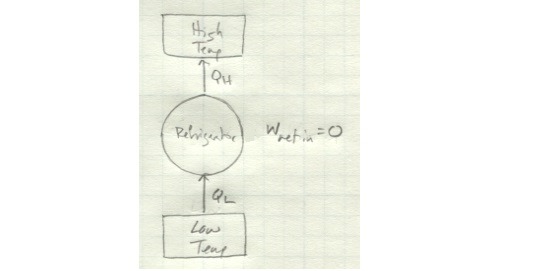
There’s no net work input here, so the energy taken in as Q_L magically increases in quality to be output as Q_H without any other energy input.
Note that all of these formulations of the second law can be expressed mathematically in the same way: Q_H is equal to the sum of Q_L and W_net.

If you have a device in which any of these terms are zero, something is wrong.
We’ve spent some time on the second law of thermodynamics - energy flows naturally from high potential to low, from hot to cold. Most of us have refrigerators or air conditioners in our dwellings - devices which seem to violate this law. They somehow move heat out of a colder space and into a hotter space. So what is going on here?
The short answer is that the refrigerant in these devices acts as a temporary energy storage medium - by some clever manipulation, we can move the refrigerant through a cycle such that at the point it travels through the space we want to cool it absorbs energy, which it needs to dump when it gets to the warmer exhaust space.
Here’s a typical refrigerator schematic.

Refrigerant enters the compressor as vapor. The compressor does work to increase its pressure. Increasing its pressure also increases its temperature, so by the time it hits the condenser it’s relatively hot. In fact, it’s hot enough relative to the surrounding environment that it sheds heat as it flows through the condenser. By the time it leaves, it’s condensed and much cooler, although it’s still at a very high pressure. It goes through an expansion valve from here, which drastically drops both its temperature and pressure. So it enters the evaporator as a low temperature, low pressure liquid. In this state, it is low enough energy that it absorbs energy from the surrounding environment going through the evaporator. When it leaves, it’s a vapor again, ready reenter the compressor and start another cycle.
This works because we are injecting energy into the cycle in the form of work done by the compressor in order to get the refrigerant to a state where the heat it absorbed from the refrigerated space can be dumped to the outside environment.
